Only quite recently historically speaking, mankind acquired the know-how to change the properties of living species: as people settled and developed agriculture. But it required a revolution, much later, to develop the knowledge to do this in a purposeful way.
Hans Tramper is professor emeritus in Bioprocess Technology at Wageningen University and reflects on the development of his subject in a series of essays. His pieces were published so far on 18 June, 30 June, 11 July, 22 July, 19 August , 10 September and 21 September 2018.
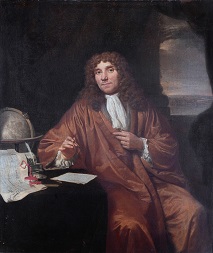
Four revolutions
The Dutch monthly Chemisch Magazine ran an article in its January 1996 edition, headed ‘New Technological Trends 21st century’ called ‘Gene therapy will cause a fourth medical revolution’. It starts as follows:
‘More than 4000 diseases and defects are caused by a defect in a single gene. Gene therapy could be the solution, even though the technology is just in its infancy. Gene therapy will cause a new revolution in medicine, so insiders believe. Earlier in history, the battle against diseases went through three major revolutions. This started when sanitary provisions and sewers were promoted, which largely did away with infectious diseases. Then came anaesthesia, that allowed doctors to treat conditions under sedation. Then the introduction of vaccines and antibiotics, that help prevent and cure many infectious diseases caused by viruses and bacteria.’
Do we engineer life in any of these so-called revolutions? No, at best we prevent and alleviate human suffering by these means, and prolong life, but in no way do we engineer human life as such, the genes. In gene therapy though, we do. A monogenetic disease is caused by a single non- or badly operative gene. Gene therapy is intended to take away this cause of the condition, and in so doing prevent or heal it. Now, after a twenty-year period of trial and error, gene therapy is still in its infancy, revolution is nowhere in sight yet. Sure, some first successes have been achieved. For instance, uniQure, based in Amsterdam, is one of the frontrunners in this area. They introduced the first approved genetic therapy to the European market, Glybera, a treatment for LPLD (lipoprotein lipase deficiency), a rare metabolic disorder. Some 30 people in the Netherlands (world-wide some 5,000) suffer from this disease, they do not or insufficiently produce lipoprotein lipase (LPL), a fat processing enzyme, because of a genetic deficiency. If it functions properly, LPL processes some 150 grams of fatty material per day that we ingest. If people have this deficiency, large chunks of fatty material keep moving around in the blood stream. These end up in the pancreas and may cause very painful inflammations there. For the time being, a diet that strictly avoids fats and alcohol, is the only remedy around. But for commercial reasons, too few customers, this gene therapy has been shelved again.
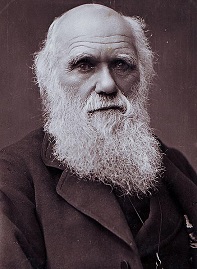
We need regulation
As for the fourth revolution, CRISPR-Cas technology is much more promising than gene therapy. It can strongly facilitate gene therapy, and moreover, with this technique we can modify DNA purposely, effectively and cheaply, and in so doing change many conditions of life. So with this technology, engineering life would seem much easier, and then I define engineering life as the purposeful modification of DNA in such a way that it will serve human and animal wellbeing, and perhaps in a wider sense better and more sustainable conditions on our planet (see Essay 1). In this light I would propose as the ultimate goal for our society: effectuate a transition from our present instable world that is clearly out of equilibrium – lending much power and wealth to a few and poverty and misery to many – to a new condition characterized by general wellbeing; a condition within the carrying capacity of the planet. This may sound presumptuous, but it corresponds to what The Royal Society voices in a video on gene technology; a video intended to inform the general public in view of debates leading to regulation as to how far we would like to go, the same goal that I have in mind writing these essays. This transition is also in line with the 17 new development goals for 2030, adopted by the UN in 2015, that address the entire global community: climate, safety, human rights and better wealth worldwide. With this difference that I do not speak of global wealth but of global wellbeing. In Essay 3 We need a transition! I will go into this more deeply, as I intend to show that staunch opponents of gene technology do not serve their proper goals, but also that its proponents still have to mount many obstacles. I also intend to argue that there should be a difference between what we can and what we should do. Technology is neutral, but people are not. We need limits and regulations, and discussions on their nature and place are required to establish them.
Towards a holistic approach
Why is this new technology so special, particularly for medical technology? Without going into detail already, we can remark that that in our lives and in our bodies, everything is tied up with everything. So far, many sciences, particularly the medical science, have looked into single factors: one single organ, one cell, one genome, one cell, in short science took a reductionistic view. This has served us well. Through simplification of the questions at hand, we discovered effective medicines, like penicillins, and developed effective instruments for feeding the world, like fertilizer and a whole array of pesticides and growth stimulants. The reductionistic approach has taken us to where we are now, the world of today. For the world of tomorrow, this will no longer do. We can no longer ignore life’s complexity, we need to develop a holistic approach. Take as our object the earth as a whole, or at least treat systems as a whole.
Evolution
The earth came into existence some 4.7 billion years ago and the first living ‘creatures’, very simple cells, started inhabiting the earth’s seas after a billion years, approximately. In the billions of years to follow, these cells evolved and about one billion years ago, the first visible multicellular organisms came to life, molluscs like jellyfish. But it was only as the first plants started to inhabit solid ground, just half a billion years ago, that the evolution sped up and many new life forms came into being. Mammals are about two hundred million years old, but humans as a species exist just two hundred thousand years. Engineering life, purposely changing DNA, was still totally out of the question.
The first proof of some scope for engineering life on a macroscopic scale was delivered by nomadic hunters who started to settle and developed primitive agriculture, some ten thousand years BC. They discovered how they could grow vegetables and grain, constructed houses and domesticated cats in order to control rats and mice that ate their yields; and cattle, goats and sheep as well, for their meat and milk. They gathered the best seeds for sowing the following year, in order to improve their yields – a kind of seed improvement in other words, but they were still far removed from engineering life as such, or understanding how this worked; the dawn of that insight still had to wait some ten thousand years, until the second half of the seventeenth century.
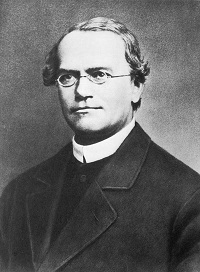
Revolutionary scientists
What would biology, particularly cell biology, be without the microscope? It is still one of the most important instruments in the biological labs. Antoni van Leeuwenhoek (1632-1723) was its pioneer. Starting from the very inadequate know-how of his time, this Delft linen merchant constructed lenses of unparalleled strength in a very smart way. With them, he studied all organic materials that he could lay his hands on, including his own. In rain water he discovered the ‘smallest animals that I have seen so far’. He called them ‘animalcules’, renamed later by Pasteur and Koch as microorganisms. His work marks the start of microbiology. From 1673 onwards, he reported his findings to The Royal Society. The original scepticism there changed into great respect after some years, and in 1680 this autodidact was appointed a member to this famous and learned London organization.
In the nineteenth century, several developments contributed to a better understanding of the essence of life. Three names need to be mentioned. First of all that of Charles Darwin (1809-1882), inextricably bound up with the evolution theory, which he published in On the Origin Species in 1859. Life on earth is in continuous change, he proposes, a process in which new species always develop from old ones, with primitive cells as the prime source. Until this day, hard-core creationists (people who believe in the story of creation) contest this. According to Darwin, all life’s manifestations are related, and built from living cells with major similarities. In the twentieth century, Darwin’s theory has been confirmed and completed over and over again, by genetic, cell biological, palaeontological and molecular biological research, and nowadays particularly by genome research. Darwin was both fascinated and frustrated by the question of hereditary characteristics: how on earth can children look like both parents, but still be different from them? A first attempt to answer this question was made in an Austrian monastery shortly before Darwin’s book was published. Ironically, Darwin did not bother to read the manuscript that this researcher sent him. Unfortunately, it took many years for those results to become recognized, till the end of that century; both researchers had passed away by that time. But we certainly need to mention the name of this researcher who was not acknowledged during his lifetime. It is the Augustine monk Gregor Mendel (1822-1884). Between 1850 and 1860 he crossbred thousands of pea plants and noticed how properties were passed on from generation to generation. From these experiments he inferred the famous Mendel laws, rules that lay the basis of classical genetics. For that reason, he is called the father of genetics, and without genetics no gene technology.
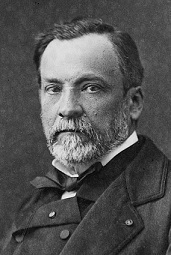
Pasteur’s discoveries
The last nineteenth century scientist to be mentioned in this connection is the Frenchman Louis Pasteur (1822-1895), a researcher pur sang, educated in chemistry and biology. Napoleon III asked Pasteur to look into the question why wine, then already one of the most important export items of France, decayed when transported to the (foreign) customer. Together with beer and bread, wine is one of the oldest biotechnological products. These date from prehistoric times and facilitated, even made more pleasant, life back then. At the time, people did not have any knowledge of the underlying processes of course, we got to know that much later, in particular through Pasteur’s research. In 1867 he discovered some awkward microorganisms that spoilt fermentation to wine and beer. His subsequent studies resulted in three simple, but oh so important guidelines for producing and storing wine, but also for fermentations in general.
The first guideline, which is a matter of course for us now, is hygiene. Wine needs to be produced and stored in clean equipment, in absence of air and the many microorganisms that move around in it, or else it will soon react to something else and decay. Often the product will be vinegar, and the purposeful production of vinegar from wine is an ancient biotechnological process as well, that employs acetic acid producing bacteria.
The second guideline consists of the application of a new process, an innovation of Pasteur’s, that carries his name, pasteurization. It builds upon the hygienic process required for product quality. When wine, beer or some other product like milk, is heated to 75oC for some time, most (pathogenic and food poisoning) microorganisms will die, which will postpone quality loss and decay resulting from unwanted fermentations for quite some time. For these two first guidelines, we can call Pasteur one of the founders of the first medical revolution.
Pasteur’s third scientific contribution consisted of understanding the role of oxygen in fermentations. If yeast is grown in an undersupply of air, i.e. oxygen, yeast cells cannot completely oxidize the available sugar to carbon dioxide, leading to the production of alcohol. An ample supply of oxygen will primarily stimulate cell growth, and will reduce alcohol production.
And there is quite another development initiated by Pasteur, the development of a virus vaccine against rabies. For this reason, he can also be called one of the founders of the third medical revolution.
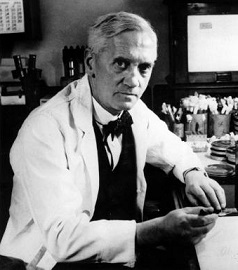
Revolution: penicillin
With regard to this third revolution, one name springs to mind first and foremost: Sir Alexander Fleming (1888-1955). In 1928, this British doctor and microbiologist discovered penicillin, through veritable serendipity. One day one of his bacterial cultures, a Staphylococcus, that he bred in petri dishes, was polluted with a mould, later identified as Penicillium notatum. Looking at it again when he was about to clean the dish, he noted that all bacteria had disappeared around the mould. It is Fleming’s major achievement that he realized the importance of this observation, i.e. that a substance – called penicillin by him, because it was produced by the Penicillium mould – stood in the way of bacterial growth. For this discovery he was awarded the Nobel Prize for Medicine in 1945. But he did not contribute much to the actual development of penicillin to a proper medicine. This was done by Florey and Chain who were awarded the Nobel Prize together with him. Penicillin has been modified to a series of substances that still belong to one of the most important classes of antibiotics. The huge promise of this antibiotic, that became apparent for the first time during the Second World War, has been fulfilled in all ways possible. The number of human lives that have been saved by this class of antibiotics in the control of bacterial infections is innumerable. And there is more. Further development of penicillin production processes has been a major stimulus in the development of modern biotechnology in general, and of large-scale biotechnological processes in particular.
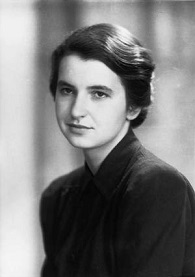
Moving on to purposely engineering the core of life, DNA, many subsequent steps still had to be made; from discovery of the substance to disclosure of its structure. The substance was discovered by the Swiss biochemist Johann Friedrich Mieser in 1869. From the discovery of the four nucleotide building blocks, to the way in which these bind together, and the discovery of the double helix structure, finally James D. Watson, Francis Crick, Maurice Wilkins and Rosalind Franklin published the correct chemical structure in Nature in 1953. In 1962 the three men were awarded the Nobel Prize for their achievements; Rosalind Franklin had passed away already then.
Interesting? Then also read:
CRISPR-Cas: a prize winning technology?
A rich harvest on genetic technology
Genetically modified food